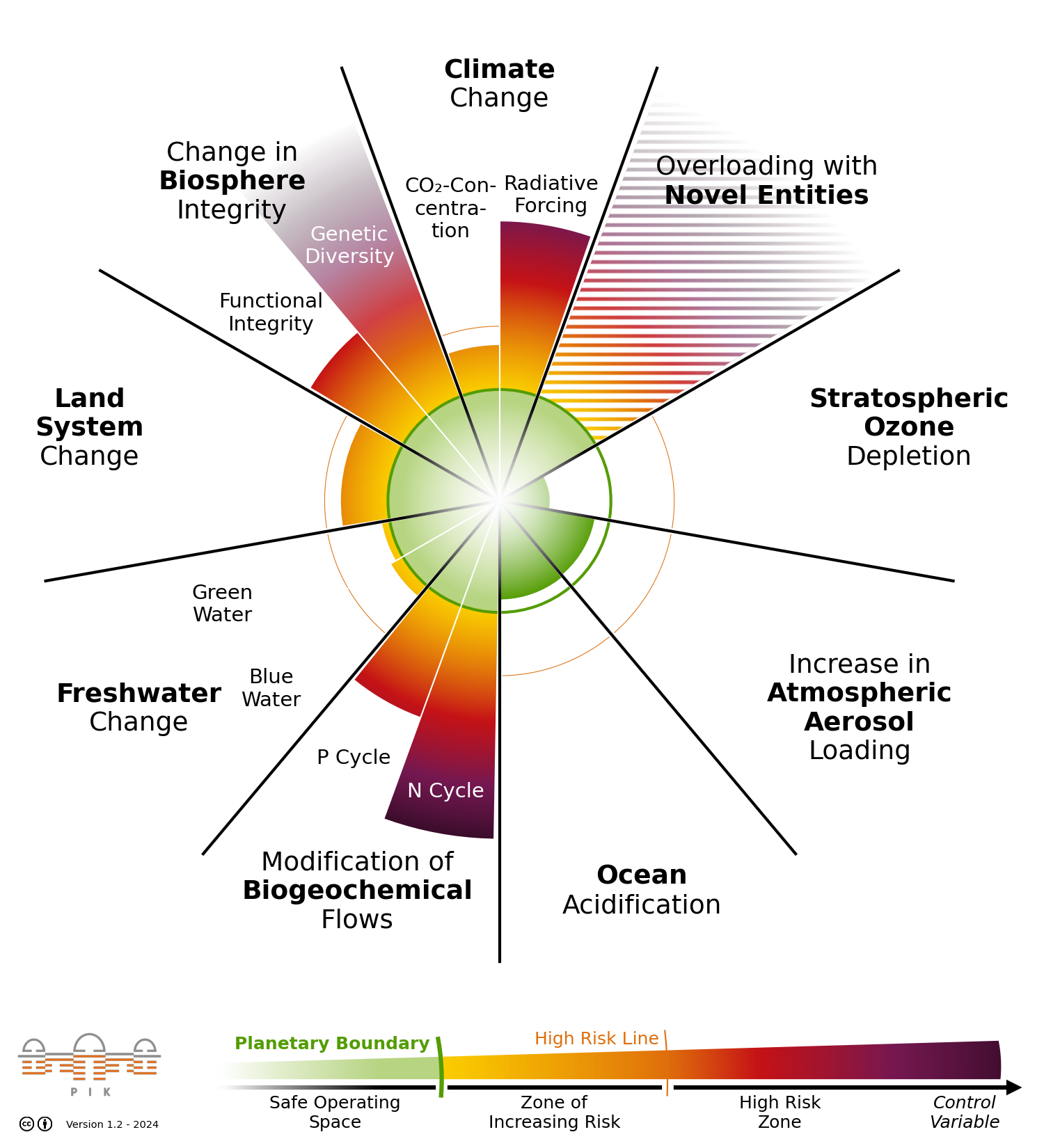
The current status of the nine Planetary Boundary systems and processes, data from Richardson et al., Science Advances (2023). The length of the wedges represents the current status with respect to the planetary boundary (green) and the high-risk line (orange). Smooth fading indicates an uncertainty range, while dashing means that there is no quantification of the current state beyond the planetary boundary. Different variants of this representation (high-resolution, colorblind-friendly) are available for download here.
Since the first and second Planetary Boundaries publications in 2009 and 2015 1,2,3, the Planetary Boundary framework has been discussed, critically assessed and enriched by countless contributions from a broad range of scientific disciplines. The latest update, from 2023 4, provides a synthesis of the most recent scientific developments, updates based on the latest scientific insights, and fills some long-standing gaps: for the first time, the status of each nine Planetary Boundary processes and systems is quantified (see press release). PIK News and Publications about Planetary Boundaries
Frequently Asked Questions
Planetary Boundaries are defined for those nine biophysical systems and processes that regulate the functioning of life support systems on Earth and ultimately the stability and resilience of the Earth system. These nine have been scientifically scrutinised since their first introduction in 2009, suggesting that sustainable stewardship, within scientifically defined boundaries, gives humanity a good chance of safeguarding the Earth system in a Holocene-like state conducive for human development. For each of the Planetary Boundary systems/processes, quantitative boundaries are set for critical control variables that are proven to be good indicators of each process' function (FAQ 3). Transgressing this safe boundary level for a control variable puts the conditions that have enabled human development and wellbeing at risk (FAQ 5). The nine Planetary Boundaries include: climate change; overloading with novel entities; stratospheric ozone depletion; atmospheric aerosol loading; ocean acidification; interference in biogeochemical flows; freshwater change; land system change and change in biosphere integrity.
The scientifically-defined boundary levels collectively define the safe operating space of the Earth system.
The term ‘Planetary Boundary’ is often used interchangeably to describe both the quantitative safe boundary level for a control variable, as well as the underlying system or process. For example, climate change is a Planetary Boundary (process), while 350 ppm is the safe Planetary Boundary (level) for climate change.
We have existed on Earth, as modern humans, for approximately 200 000 years. This means we have evolved, with the same physical and intellectual capacity as today, through two Ice ages (approximately 100 000 years long) and two warm inter-glacial periods (the Eemian some 110 000 years ago and the Holocene, our current inter-glacial state of the Earth system). During the bulk of this period, we humans survived on Earth as hunter-gatherers. We were a few million people, scattered and living semi-nomadic lives, until we left the last Ice Age, some 16 000 years ago, and entered the most recent inter-glacial, the Holocene, approximately 12 000 years ago.
The Holocene is (or was) an extraordinarily stable state of the Earth system: temperatures on Earth stabilised at 14±0.5°C. Ecosystems, precipitation patterns, seasons and temperatures settled within narrow "life-supporting" ranges, providing us with the states of the biosphere, hydrosphere, and cryosphere of the Earth as we know it. Earth had barely settled in this stable Holocene state, when we went through the Neolithic revolution, domesticating plants and animals simultaneously across continents on Earth some 10 000 years ago. We became farmers, living in sedentary communities. This was the starting point of civilisations as we know them today. The conclusion, a foundation of the Planetary Boundary framework, is that the Holocene state of the planet is the only state of the planet we know for certain can support the modern world as we know it.
This gives the Planetary Boundary framework a reference to measure against. The questions that the framework attempt to answer then become: (1) what are the biophysical processes and systems that regulate the state of the Earth system? (we have identified and confirmed nine, see FAQ 1) And, (2) how far can each of the boundary processes/systems be changed before we risk moving the life-support systems and the stability of the Earth system away from Holocene conditions (FAQ 3)?
Using the Holocene as a reference point can wrongly be interpreted as if the Planetary Boundaries try to imply a return to the Holocene. This is not the case. It is impossible. We cannot, of course, envisage a transformation back to a "virgin" state of the planet, with 280 ppm of CO2, and zero transformation of terrestrial ecosystems into managed land (agriculture).
This is why we use the term "Holocene-like". The reference point for the search of quantified safe Planetary Boundaries is to answer the following question (for each control variable, see FAQ 3): how much stress does a Planetary Boundary process/system tolerate before reaching significant risks of deviating away from a manageable Holocene-like interglacial state? This is why, for example, the climate planetary boundary is 350 ppm (equivalent to some 0.4°C of global warming, i.e., within the 14±0.5°C range), not at the "virgin" 280 ppm level.
For each of the nine Planetary Boundary processes, one or more control variables are defined. A control variable is (ideally) the dominant parameter explaining the state of the planetary boundary system in question. For example, Carbon Dioxide Concentration is one of the control variables used for climate change, while land use change is measured via the proportion of the globally remaining original forest cover. A control variable can also be a proxy indicator for the state of the planetary boundary system/process, selected based on our scientific understanding and availability of global data. For example, extinction rate is used as a proxy indicator for genetic diversity, one aspect of biosphere integrity. Methodologically, the scientific assessment of each control variable results in an uncertainty range (see FAQ 5). For more detailed information, please consult the most recent PB update 4.
The control variables allow to numerically pin down two important points for each of the nine PB processes:
- The value of the Planetary Boundary - the safe level, which is at the outer boundary of the safe operating space (the green circle in the figure above). These values are determined through a variety of methods. Essentially it is a process of risk assessment grounded in scientific modelling, summarising published research, and expert elicitation: The most up-to-date scientific knowledge is used to determine the level of change that: (a) would represent a significant departure from the conditions that prevailed throughout the last 12 000 years (the Holocene, FAQ 2 and 2a), and (b) would risk destabilisation of the respective process, erosion of its functions at regional to global scales, and potentially regime shifts - all with the risk of reinforcing feedbacks or interactions.
The value for each Planetary Boundary is chosen according to the precautionary principle: The Planetary Boundary level is set at the lower end of the assessment scientific range of uncertainty (see FAQ 5). For example, in the case of climate change the uncertainty range (for the control variable “CO2 concentration”) is estimated at 350-450 ppm CO2. The Planetary Boundary framework sets the safe boundary at the lower end of the uncertainty range in science, i.e., at 350 ppm. - The current status of the Planetary Boundary processes and systems is identified by combining the most recent data and modelling results. In the figure above, the current status is represented by the length of the wedges, with respect to the Planetary Boundary level (green circle in figure above) and the high-risk line (thin orange line).
No.
Tipping points are thresholds beyond which certain critical, large-scale components of the Earth system (tipping elements) can be tipped into a qualitatively new state, often characterised by far-reaching changes to conditions on Earth (see PIK infodesk: Tipping Elements).
Planetary Boundaries, on the other hand, are demarcations for where various risks (see FAQ 3) rise above a safe level. Such a risk may rise in a smooth and gradual way, without any thresholds. This does not exclude, however, that in certain cases the Planetary Boundary is set to avoid reaching a tipping point. In the case of climate change, the Planetary Boundary level was chosen to ensure that the global temperature change compared to pre-industrial levels stays well below the tipping points for known tipping elements (see FAQ 5).
So in short, transgressing a Planetary Boundary does not mean crossing a tipping point. But, nevertheless, tipping point science is one source of evidence used in setting the safe boundary quantifications: This means that Planetary Boundaries are set to avoid crossing tipping points anywhere in the Earth System. Consequently, tipping points play a role for both the Planetary boundaries with evidence of tipping point behaviour (e.g, the climate system), and boundaries without such evidence (e.g., the hydrological cycle), because the processes are interlinked: major changes in green and blue water, for instance, have impacts on biomass production (biosphere integrity), which in turn has impacts on the climate boundary. This means that non-linear changes and tipping point dynamics (when feedbacks change from negative to positive, causing (often) irreversible state shifts), is used to determine safe boundaries for “non-tipping point” boundary processes as well.
Once a Planetary Boundary is transgressed, the process or system in question enters a zone of increasing risk (also called “zone of uncertainty” or “danger zone”). Here, risks of changes increase, but the scientific evidence is scattered. This uncertainty minimises as we enter the high-risk zone, where there is scientific evidence that the probability of damage becomes high. Transgressing a boundary, and moving into the “danger zone” or even the “high-risk zone”, does not imply immediate catastrophic change. We are rather running an ever-higher risk of fundamentally destabilising the life support system on Earth, and, in the long term, the stability and resilience of the Earth system, which would manifest in far-reaching environmental damage.
Six of the nine Planetary Boundaries are currently transgressed. That is the case for climate change; overloading with novel entities; modification of biogeochemical flows (nitrogen and phosphorus cycles); freshwater change (blue and green water); land system change and change in biosphere integrity.
Three of the Planetary Boundaries have been transgressed to such an extent that the high-risk zone has been reached (for climate change, modification of biogeochemical flows and change in biosphere integrity).
We are still in the safe zone in three cases: the increase in atmospheric aerosol loading, ocean acidification (where the Planetary Boundary is fast approaching), and stratospheric ozone depletion. Stratospheric ozone depletion was regionally transgressed (over Antarctica), but through concerted scientific, policy and industry efforts we have been able to bend the curve, and the Ozone layer is slowly re-establishing itself. This turnaround can be directly attributed to the success of the Montreal protocol, with which an international agreement was met to regulate the use of nearly 100 man-made ozone-depleting substances, including CFCs.
The recent assessment of Earth system boundaries (ESB’s, Rockström et al, Nature, 2023) builds upon the Planetary Boundaries (PB) framework, and integrates other existing ideas like doughnut economics and the Sustainable Development Goals. The two key differences are that (a) the ESBs define a safe and just operating space, and (b) focus on scaling of quantitative boundaries from local to global level.
The ESB's have been advanced by the Earth Commission, a scientific initiative to establish a global science assessment mechanism for safe and just boundaries. In this sense, Planetary Boundaries science is one source of evidence for the broader assessment of ESB's.
By integrating justice, the aim is to avoid significant harm to people, like loss of lives, livelihoods or income; displacement; loss of food, water or nutritional security; and chronic disease, injury or malnutrition. To this end, eight control variables (for safety and justice) have been identified for five of the Planetary Boundary processes (climate, the biosphere, fresh water, nutrients and air pollution by aerosols). These eight are displayed in the published ESB figure (1 for climate change, 2 for biosphere integrity, 2 for nutrients/biogeochemical flows, 2 for freshwater change and 1 for aerosol loading).
The control variables (which are mostly different to the PB control variables) are chosen to allow for an operationalisation of the approach down to regional and even local level, striving to provide the basis for on-the-ground applicable science-based targets (SBTs), as envisioned within the Global Commons Alliance.
The nine Planetary Boundary processes remain the established reference and a suitable starting point for assessments like the ESB’s: while in each of the boundary systems/processes multiple valid perspectives or foci are thinkable, these nine have proven to comprehensively cover the relevant dimensions of a safe operating space for humanity on Earth.
References
1. | Rockström, Johan, Will Steffen, Kevin Noone, Åsa Persson, F. Stuart Chapin, Eric F. Lambin, Timothy M. Lenton, et al. 2009. ‘A Safe Operating Space for Humanity’. Nature 461 (7263): 472–75. https://doi.org/10.1038/461472a. |
2. | Rockström, Johan, Will Steffen, Kevin Noone, Åsa Persson, F. Stuart III Chapin, Eric Lambin, Timothy Lenton, et al. 2009. ‘Planetary Boundaries: Exploring the Safe Operating Space for Humanity’. Ecology and Society 14 (2). https://doi.org/10.5751/ES-03180-140232. |
3. | Steffen, Will, Katherine Richardson, Johan Rockström, Sarah E. Cornell, Ingo Fetzer, Elena M. Bennett, Reinette Biggs, et al. 2015. ‘Planetary Boundaries: Guiding Human Development on a Changing Planet’. Science 347 (6223): 1259855. https://doi.org/10.1126/science.1259855. |
4. |
Richardson, Katherine, Will Steffen, Wolfgang Lucht, Jørgen Bendtsen, Sarah E. Cornell, Jonathan F. Donges, Markus Drüke, et al. 2023. ‘Earth beyond Six of Nine Planetary Boundaries’. Science Advances 9 (37): eadh2458. https://doi.org/10.1126/sciadv.adh2458.
|